If you’ve sat down and played a Pokémon game any time since the year 2000, chances are you’ve run into an egg. In these eggs you can find any type of Pokémon, even a rare Legendary. In many of the recent games, like Sword and Shield, you can leave Pokémon at the nursery, walk or bike around for a bit and BOOM – an egg will be ready for you to hatch your very own Pokémon.
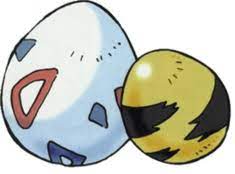
Pokémon Eggs are pretty artsy from the start. image – The Pokémon Company
But what might the Pokémon actually look like inside that egg? And what can that tell us about our own development?
That’s where the science comes in. While an organism is in the early stages of development (before it is hatched or born), it’s called an embryo. For animals that develop inside an egg, different structures could be present in the egg depending on the species that laid it. Birds and reptiles are called amniotes–they develop in eggs that have hard external shells for protection and a membrane called an amnion which protects them from drying out. These types of eggs also have a recognizable yolk, which supplies nutrients to the growing embryo. These look much more like what we’re used to in the world of Pokémon.
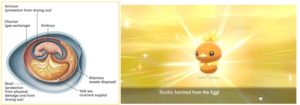
Diagram: Introductory and General Biology, Torchic, The Pokémon Company
Although all the eggs in Pokémon look like this, in real life, different types of animals hatch from different very different types of eggs. Amphibians, like frogs, toads, newts, and salamanders, are slick-skinned animals that need to live near water to survive. Amphibians and fish develop from jelly-like blobs that normally are found in ponds and lakes. If you were hatching something like Mudkip, Palpitoad, Froakie, or Poliwag in the real world, their eggs would probably look more like this:
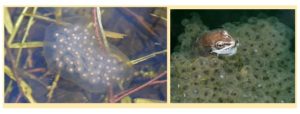
Spotted salamander eggs (left) and wood frog eggs (right)
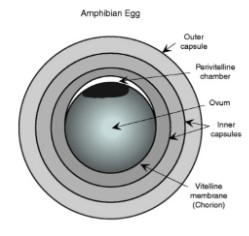
An amphibian egg, Diagram by Vitt (2008)
Each black dot you see is the egg itself–called an ovum– surrounded by a gelatinous substance made up of different layers that holds the eggs together in the water or on a branch. This may surprise you, but that little black egg is actually a single cell that is shockingly visible to the naked eye. If the conditions are right for the embryo to develop, this cell will start to divide and begin to form the amphibian embryo. This may not be the case for every type of amphibian, but it can give you a general idea of how eggs from different species of animals might look (and develop) differently than the classic egg we get while playing Pokémon.
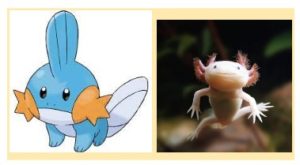
A Mudkip and an axolotl – cousins? images: The Pokémon Company, National Geographic
Now imagine we have two different Pokémon eggs in front of us: a Torchic egg, which would be similar to a chicken, and a Mudkip egg, which would be similar to a salamander with visible gills, like an axolotl. (Sorry Treecko–not every Gen 3 starter could make it into the article). Let’s take a closer look at the developing embryos and see if there’s something we can learn about ourselves.
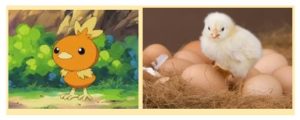
Torchic and a real chick. similar bodies means similar development? images: The Pokémon Company, LiveScience
If the animal has a spinal cord, it’s called a vertebrate. Fish, reptiles, amphibians, birds, and mammals are all vertebrates, so we can reasonably assume a Torchic and a Mudkip would be vertebrates too. All vertebrates have a similar body plan, which is a set of characteristics that are common in similar animals. Some of the characteristics can include the type of symmetry we see in the body shape, the number of tissue layers, the presence of a body cavity, and other things we see during development. Even though salamanders and chickens don’t seem very similar to us, their body plan looks similar to each other and other vertebrates. One example of these similarities is in the symmetry of the organism’s body. Both a chicken and a salamander have bodies with bilateral symmetry, meaning that if you drew a line down the middle, each side would look like a mirror image of the other.
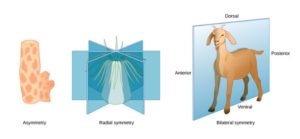
The types of symmetry; image – Pearson Education (2009)
Invertebrates, like a Sea star (or a Staryu), do not show bilateral symmetry. Instead, they show radial symmetry, which refers to symmetry around a central axis. When you look at these kinds of characteristics, it makes sense that animals that seem different (like a salamander and a chicken) might have more in common than we think.
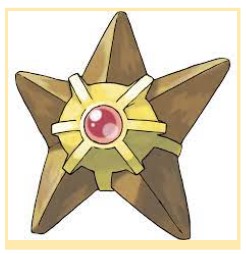
The Mighty Staryu; image – The Pokémon Company
Now here’s the weird part: even between animals that look nothing alike in adulthood, their embryos look astoundingly similar early in embryonic development. Don’t believe it? Take a look at this image and see for yourself. The less developed embryos are in the top row, whereas the most developed are in the lowest row.
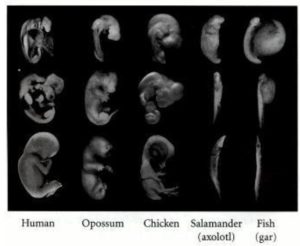
Photograph by M. Richardson, retrieved from Developmental Biology. 6th edition.
In the earlier stages of development, you can see that all of the organisms have the same general shape. If you were looking at a Torchic and a Mudkip developing in their Pokémon eggs, all you’d probably be able to see is the head and the tail compared to the rest of the body. It also looks like their skin might be similarly smooth, because you can’t see any bird feathers on the chicken embryo yet. What you can’t see are even more similarities– all vertebrate embryos have spinal cords, early kidneys, and structures called pharyngeal arches. In these early stages of development, a Torchic and a Mudkip would be incredibly difficult to differentiate (unless you happened to be Professor Oak.)
As they proceed through development, they begin to look more and more different as the initial tissue differentiates into tissue with a specific function. Let’s take limb development as an example. In vertebrates, early limb development is essentially the same–again, if you just took a photo of the embryo like the one shown above, you wouldn’t see much of a difference between the Torchic and the Mudkip. In early development, both would have limb buds–protrusions of tissue that come from the embryo. As the Pokémon develops in the egg, the limb buds would slowly elongate and begin to differentiate into legs, arms, or wings. Mudkip would probably have four limb buds, which develop into the four similar-looking legs he stands on. The Torchic would have four limb buds too, but two of them would develop into wings and two of them would develop into legs. You can see how different the arrangement of the bones looks once the limb bud has developed into the more specialized limb.
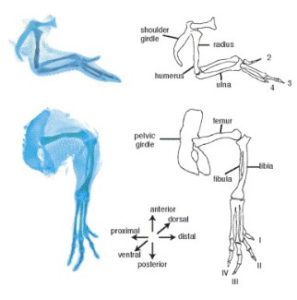
Chicken wing structure (above) Chicken leg structure (below)
images – C. Tickle
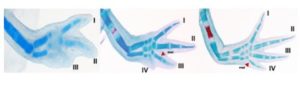
Axolotl limb; image – Purushothaman et al. 2019
(They look blue because scientists used a blue chemical stain in order to get a better look at the limbs– not because they are from Water-type Pokémon!)
One key concept to keep in mind is that structure determines function–meaning that the way a body part is structurally organized allows it to be used by the organism for a particular purpose. Think about the shape of the Torchic’s wing versus the shape of the Mudkip’s legs. Which limb shape might be better at a flying-type move, like Aerial Ace?
If you thought Torchic, you thought correctly. Although Torchic is a Fire-type Pokémon and not a Flying-type, its wings would certainly be better at flying type moves than the short, stubby legs of Mudkip. As Torchic grows into his more developed forms, Combusken and Blaziken, those wings continue to grow and he can learn even more Flying-type moves.
Another example of structure and function would be the development of breathing organs like lungs or gills. Gills are used by water dwelling animals to breathe for extended periods of time while submerged in the water. Both lungs and gills develop from an initial, undifferentiated protrusion of tissue. In animals that develop lungs, this tissue is called a “lung bud”, but is called a “gill bud” in animals that go on to develop gills. Would a Torchic or a Mudkip be better at a move that requires them to be underwater for a long amount of time, such as Dive?
Definitely Mudkip. His orange, fan-like gills would allow him to be underwater for much longer periods of time compared to lungs like Torchic’s or ours. From these examples, you can start to see how different structures are better at executing different functions–functions that make each animal better in their particular environment.
What about us? We also start out with four limb buds that give rise to our arms and legs. Our arms develop fingers that allow us to do things like hold, write, eat, or grasp a video game controller. We develop lungs from lung buds that allow us to breathe air or to let out a sigh of relief when we finally get past the Rock-type gym with a Fire-type starter. But isn’t it strange to think you once looked similar to how a Torchic or a Mudkip probably would’ve looked in their Pokémon eggs?
Additional Resources:
Comparative Embryology: The Vertebrate Body
References:
Casale J, Giwa AO. Embryology, Branchial Arches. [Updated 2021 Aug 11]. In: StatPearls [Internet]. Treasure Island (FL): StatPearls Publishing; 2022 Jan-. Available from: https://www.ncbi.nlm.nih.gov/books/NBK538487/
Gilbert SF. Developmental Biology. 6th edition. Sunderland (MA): Sinauer Associates; 2000. Comparative Embryology. Available from: https://www.ncbi.nlm.nih.gov/books/NBK9974/
Introductory and General Biology. (2020, October 4). https://bio.libretexts.org/@go/page/11539
Kerney R. (2011). Embryonic staging table for a direct-developing salamander, Plethodon cinereus (Plethodontidae). Anatomical record (Hoboken, N.J. : 2007), 294(11), 1796–1808. https://doi.org/10.1002/ar.21480
Lever, A. (2021, June 30). The Frog Life Cycle for Kids. National Geographic Kids. https://www.natgeokids.com/uk/discover/science/nature/frog-life-cycle/
Pearson Education (2009). Biology: Exploring Life (5th ed.) [E-book]. Prentice Hall.
Purushothaman, S., Elewa, A., & Seifert, A. W. (2019). Fgf-signaling is compartmentalized within the mesenchyme and controls proliferation during salamander limb development. eLife, 8, e48507. https://doi.org/10.7554/eLife.48507
Tickle C. (2004). The contribution of chicken embryology to the understanding of vertebrate limb development. Mechanisms of development, 121(9), 1019–1029. https://doi.org/10.1016/j.mod.2004.05.015
Vitt, L., & Caldwell, J. (2008). Herpetology: An Introductory Biology of Amphibians and Reptiles (3rd ed.). Academic Press.
Wolpert L. (1999). Vertebrate limb development and malformations. Pediatric research, 46(3), 247–254. https://doi.org/10.1203/00006450-199909000-00001