The moon shines at night, but some parts of it shine better than others – the retroreflectors we left behind.
Okay – quick physics refresher. When visible light hits things it will do, general one (or combos) from the following list:
Light can be absorbed: The energy contained in the light hits the atoms of the material with just the right energy and just the right way to “excite” them and make them move faster. Particles moving faster means that the temperature of those particles (and material) will increase. That light’s story is done.
Light can reflect: The incoming light hits the material, and some (or all) of it is reflected, sent back out and away from the material. The type of reflection depends upon the surface – a flat, mirrored surface will reflect back virtually all of the incoming light without changing the frequency (the color) at all, while irregular surfaces can produce a diffuse reflection, reflecting only certain wavelengths, or colors. As your local neighborhood physics teacher loves to say, we don’t see the “thing” we’re looking at itself, we see the reflection of the light bouncing off of the thing.
Light can scatter: Sometimes lumped in with reflection, scattering is different in that the light must be absorbed and then re-emitted by the material. Its frequency can change as a result of the interaction with the material, and it will be sent back out in any number of random directions. Different types of scattering can show different effects, such as Rayleigh scattering, which is why the sky is blue, although it is illuminated by the white light from the sun.
Light can diffract: Given the wave-like properties of light, it can “bend” around the edges of an obstacle or travels through a slit in material, the opening of which is less than a wavelength of light.
Light can refract: If the material is transparent, or semi-transparent to light, the light will change speed (ever so slightly) as it passes through and changes its direction relative to the direction it came in with. The most common example of refraction is the “weird” effects seen with objects partly in and partly out of water – a pencil in a glass of water for example.
Let’s focus on reflection, and what it has to do with some cool stuff we left on the moon.
How We See the Moon
We see the moon the same way we see anything – it reflects light. The main type of reflection provided by the moon is diffuse reflectivity, that is, the incoming light (from the sun) is reflected back in virtually every direction. That’s in contrast to specular reflectivity, which is seen with mirrors and smooth or polished surfaces. The moon, not being polished or smooth sends light from the sun back out every which way, making it appear to glow.
At least from our perspective.
In terms of objects in the solar system, our moon isn’t very “bright.” The measure of the light scattered by the moon – called albedo – is less than that of the earth, which can result in pictures like this – both the sun and moon were illuminated by direct sunlight.
We think of the moon as bright and white thanks to both the contrast of it being in the dark sky, and its relative closeness to the earth. Think of pictures of astronauts on the moon – the lunar soil, called regolith – is gray. Ever see an astronaut’s suit after it’s been worn on the moon? It’s dusty and dirty, and most importantly, all of the bright white outer material of the suit is now dark gray.
All the moon can do – with its surface that has the reflective color of well-used asphalt – is take the sunlight hitting it and send it back out in no particular direction. For the couple of hundred thousand years we’ve been around, we’ve looked at it and said, “Ohh…pretty” or any number of other exclamations in countless languages.
Yeah, but what does this have to do with stuff we left behind on the moon?
The Useful Stuff
Along with the lower half of their Lunar Modules, Lunar Roving Vehicles (moon buggies), tools, instruments, waste, golf balls, a picture of Charlie Duke’s family, and other weird stuff, American astronauts left behind three arrays of retroreflectors. Russians have done the same thing twice, as part of the Lunokhod program. The first was placed as part of Apollo 11 in 1969, and the last as part of Lunokhod 2 in 1973.
Retroreflectors are special types of reflectors that you’re already familiar with. Picture the reflector on a bicycle wheel (or on a whole bike) – bright, right? The video below shows the Lumen bike, which is coated with retroreflective paint. Check out around 0:36 or so – they positively glow in a Tron-like way.
The brightness of retroreflectors is due to their ability to reflect the source of illumination back, virtually on itself. It’s like shining a flashlight into a mirror at a 90-degree angle. The light is reflected at the source, rather than at an angle, say, off to the side. Like all reflections, retroreflectors need a source of illumination to reflect – they don’t produce light by themselves.
Structurally, basic (not talking about the tapidum lucidum in cat’s eyes and other animals that demonstrate “eyeshine” – the tissue is a retroreflector on a curved surface) retroreflectors are two or three reflective surfaces, all at 90-degree angles from one another. In the case of three surfaces, think of the corner of a cube made of mirrors.
In a way of thinking, these retroreflectors “catch” the light, reflect it from one surface to another, and then another. The light is reflected in the same plane, and combined with the orientation of the surfaces, the light is reflected directly back at its source. LIke this:
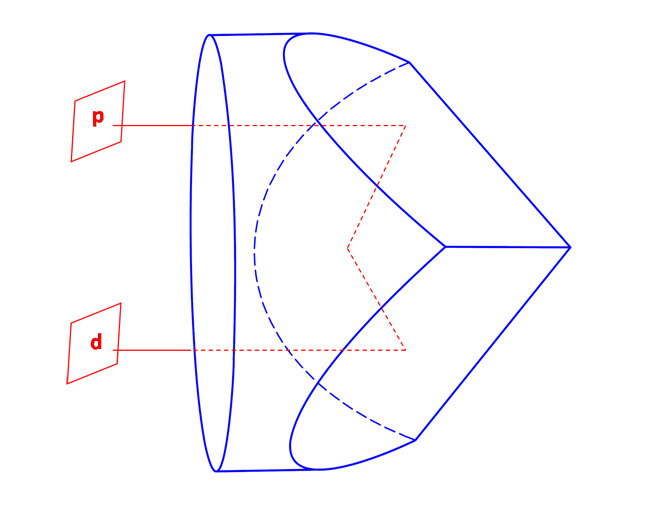
Think of this as a chunk of a glass cube, cut out of the corner. The light comes in, bounces off 3 surfaces, and then heads back out towards the source. (c) Fuzhou Laserstates Electronics Co., Ltd.
The NASA retroreflector arrays are located in a rough triangle near the Apollo landing sites that placed them, while the two Lunokhod arrays are further north and east, respectively, of the three Apollo arrays.
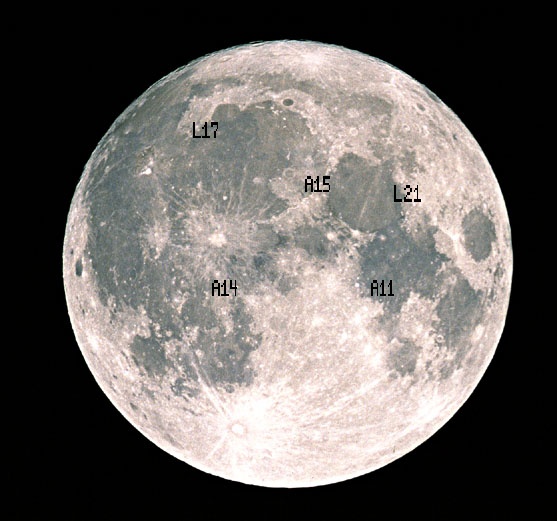
“A” are Apollo arrays, “L” are Lunokhod By NASA – http://science.nasa.gov/science-news/science-at-nasa/2004/06may_lunarranging/
Two more were to be placed by the unmanned Israeli Beresheet lander and the Indian Vikram lander, but both missions experienced hard landings (crashes), and as a result, their arrays were not placed properly. The MoonLIGHT array was scheduled to be placed near the lunar South Pole later this year as part of the MX-1E lander mission, part of the commercial Moon Express missions – but the mission has been canceled.
So for now, we’ve got five of them on the moon, between 47 and 50 years old. And they still (mostly) are functional.
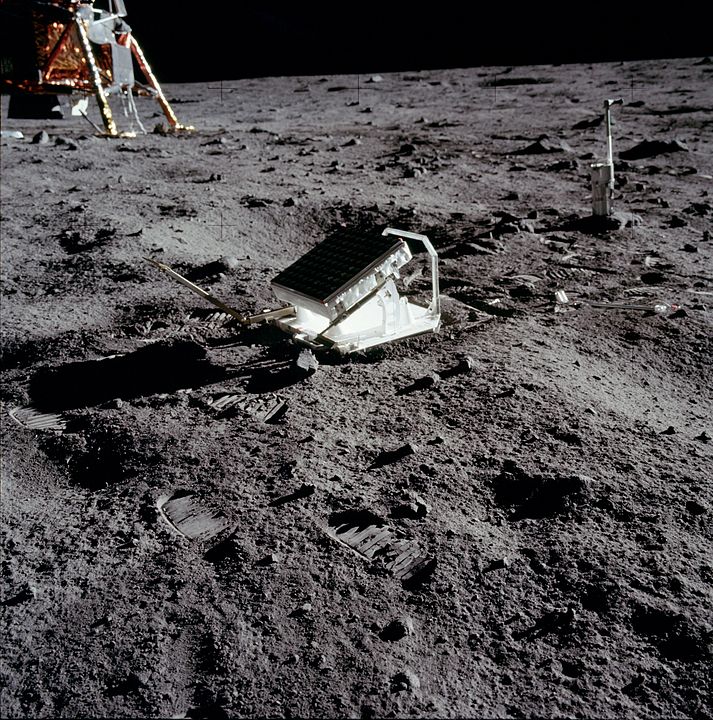
The Apollo 11 Lunar Ranging Array of retroreflectors.
So – what do we do with them, and who uses them?
Shining a Light at the Moon
Given the properties of a retroreflector as discussed above, the retroreflector arrays left on the moon’s surface can be used to precisely measure the distance between the earth and the moon. Ath Apollo retroreflectors were left on the surface specifically for this purpose, as part of the Lunar Laser Ranging Experiment. Of the arrays, Apollo 15’s is the largest, and has been used most often, but as technology and lasers have gotten better, the smaller arrays have come back into use as well.
The concept for ranging is essentially the same for ranging with radio waves (RAdio Detection And Ranging – RADAR), sound waves (SOund Navigation And Ranging – SONAR) and other laser applications (LIght Detection And Ranging – LIDAR): beam a signal with a known velocity at a target, receive information about the signal’s reflection back to the source, and then calculate its distance from the source.
To make an approximate calculation, a simple formula can be used:
Distance = (speed of light x time between signal emission and reflection detection)/2
It’s basically the same for any of the other methods used for ranging, just replace the speed of light for the speed of sound (the speed of radio is the same as the speed of light – 3.0 x 108 meters/second – “speed of light” just sounds sexier).
The fundamentals of the formula come from the most basic of physics formulas: speed.
Speed = distance/time
Solve that equation for distance, and then you get:
Distance = speed x time
For ranging, you have to divide by 2, because the signal goes out, hits the target, and then is reflected back. If you don’t divide by 2, you end up with twice the distance to the target.
The time for light to leave the earth, reflect off the retroreflectors on the moon (the actual “time of reflection” is negligible), and return to the earth is about 2.5 seconds. Do the math, and you get around 375,000 kilometers or about 233,014 miles. That’s a good approximation.
The average, center-to-center distance between the earth and moon is about 239,000 miles – it changes up and down due to a number of different factor such as the relative motion of the earth and the moon, relativistic effects, polar motion, weather, the motion of the earth’s crust, tides, the wobble of the moon – side to side and up and down and a number of other factors.
Light Out and Light Back
To actually use the retroreflectors, it’s useful to have a laser. A really, really big, powerful laser. No – that laser pointer won’t work. Think big observatory. Cannon-sized. Like the one at the Apache Point Observatory in New Mexico, which operates the Apache POint Lunar Laser-ranging Operation (APOLLO).
With the telescope at Apache Point, the distance between the earth and moon can be calculated between a few millimeters. Millimeters.
The quick version is that a high-powered laser sends pulses of monochromatic laser light at the retroreflector location. Each pulse lasts 100 picoseconds (a picosecond is a trillionth of a second). The light goes out to the moon, ideally hits the retroreflector array, and the reflection (the returning light) is collected by the 3.5-meter telescope. Sounds simple, right?
Thing is – despite what you may have learned about energy beams traveling through space from The Force Awakens’ Starkiller Base, beams of energy like lasers spread out as they travel. A lot. The narrow laser beam that leaves from Apache Point is roughly 4 miles in diameter when it reaches the moon. All of the energy of the initial pulse is spread out over that area. At this point, it helps if you think of light as particles – photons. The beam has a set number of photons, related to how much energy it had when it left (which is related to how much energy it took to create it) – now, those photons are all spread out, hitting the moon over the general region of where the retroreflector is. Sometimes, conditions are great, sometimes, not so much.
Okay, numbers: the outgoing beam has roughly 1017 photons in it. Some of those photons hit gas or water molecules in the air, dust particles or other obstacles. So not all from the pulse are getting to the moon to (hopefully) hit the retroreflector. Those that do, head back towards the source, but some will hit those obstacles their pals ran afoul of on the way to the moon.
So – total?
For each laser pulse out, approximately one photon is captured by the telescope looking for the reflection. One photon comes back to the source after reflecting on the retroreflector. We know they’re not just random photons being captured due to the monochromatic nature of the light – a means by which we can check the returning photon’s ID. Naturally, more than one pulse is sent, but still – that’s a horrible return on investment.
But we can detect it.
By the way, human eyes can detect a single photon of light, but it’s beyond faint. As described by one of the researchers who made the finding, it’s not quite a visual sensation, but more of something tickling your imagination.
Nothing against your eye, but it’s no 3.5-meter telescope hooked up to a computer that figures out the distance between the earth and the moon to the millimeter.
Oh, and as a result of the work of the Lunar Laser Ranging Experiment, we’ve confirmed (among other things) the hypothesis that the moon is getting farther away from the earth – by about 3.8 centimeters per year.
This is cool stuff.
Getting Your Retroreflector On
Making a retroreflective surface isn’t a big deal at all. Forrest Mims explains how to make one, both with glass beads as well as mirrored surfaces over at Make, and there are all types of retroreflective tape at Amazon that you can play with.
And what got me walking down this road in the first place was Adam Savage’s terrific video of making a retroreflector array as one of the one-day builds he’s been posting since the lockdown began. Check it out below. If you’re interested in making your own – or a version and need the parts he uses, he links to them in the description of his video.
As a science teacher, I’ve got my summer project set for me. I don’t want to start up the year (however we start the year) in my physics class without one!